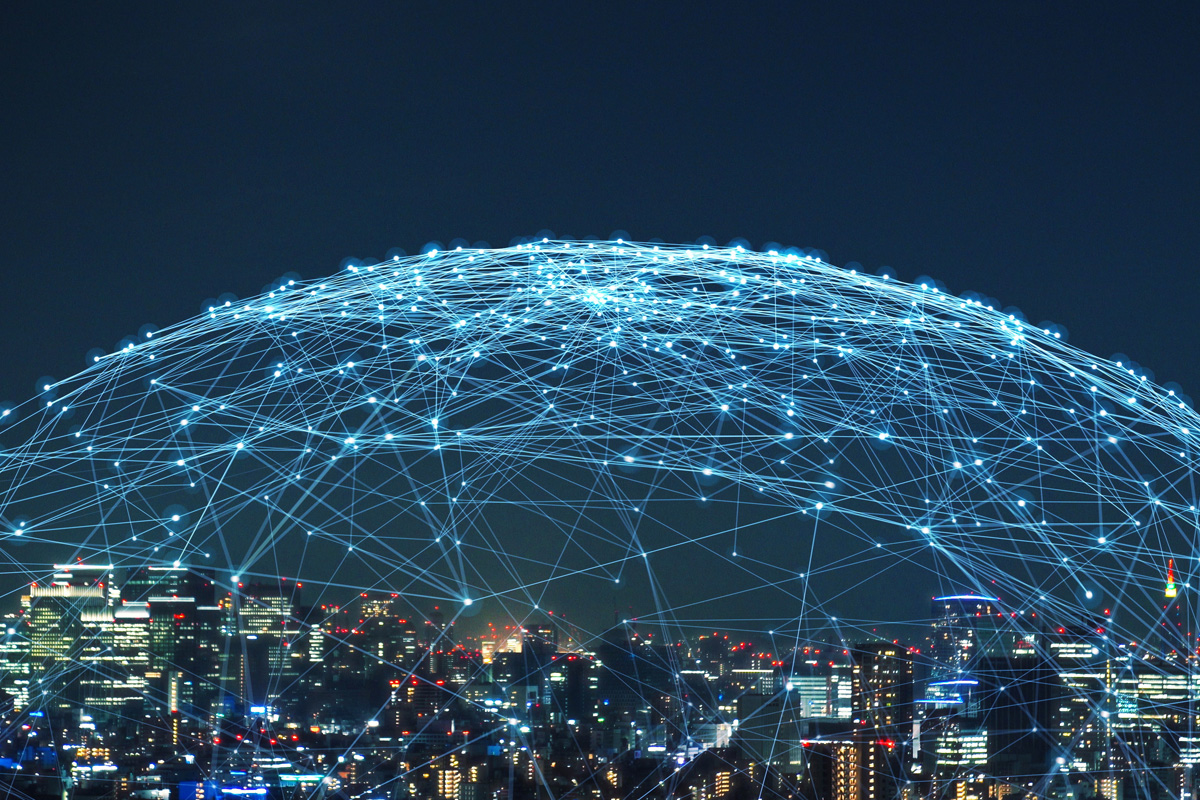
Cellular Frequency Bands and their Impact on Telematics
On episode 2 of Well’s Words, Positioning Universal CEO Mark Wells briefly discussed cellular frequency bands that smart phones, as well as mobile IOT devices, use to transmit data through the network. Today we are going to dive further into cellular frequency bands and what they mean in the greater telematics picture.
In the episode, Mark Wells illustrated how mobile IOT devices have 1-3 “bands” versus smartphones that have over 30 of these bands to work on. Likewise, he mentioned that smartphones can use multiple technologies whereas mobile IOT devices usually only use 1 technology (4G LTE). So, the question is what are these “bands” and how do they work? Let us examine this question through the lens of the smartphone.
Cellular Frequency Band Basics
Before we jump into the nuts and bolts of frequency bands and how they work, let us take a step back. Smart phones are so great because they merged the ability to makes calls with the ability to access the internet. On top of that, with a smartphone, you can access the internet from anywhere, unlike your computer. Normally, accessing the internet requires an ethernet cable or Wi-Fi connection. But as seen from walking a little bit away from your connection (say your house, for example) the connection through Wi-Fi gets lost as it only stretches a limited distance.
The G’s (Generations) of Signal
The beauty of smartphones is that devices can access the internet without the need for Wi-Fi or an ethernet cable. Smartphones can connect anywhere there is a cellular connection. 3G, 4G LTE, and 5G are all different versions of this connection, or technology as Mark Wells refers to it. 5G is the fastest connection to date, but smart phones can still use the other technologies to try and connect to the internet if necessary. This is why sometimes in bad service areas your phone shows 3G service because the 4G and 5G connections do not work there. These technologies each represent the figurative tunnel/pipe that data can travel through to get to the cell tower. More on this later.
The Wave
Data travels and is communicated through radio signals. Whenever a signal is transmitted, it travels as a waveform. Each wave has a specific frequency that distinguishes it from another wave. The wave’s frequency is how many cycles of that wave are completed per second of time and is measured in Hertz (Hz). The diagram below shows how a different frequency affects the shape of the waveform. Notice how the bottom wave completes two full cycles while the top wave only completes one.
Using this example, at a high level a cellular frequency band is a range of different frequencies. When radio signals are sent out, they actually use a range of frequencies to communicate their message. The width of that range represents the “bandwidth” of the data and indicates how much data can be processed through the signal. The more bandwidth, the more data that can be processed, leading to less congestion in the network, and thus faster speeds. As an analogy, think of the bandwidth as the number of lanes on a highway. The more lanes, the faster the traffic will flow, where the traffic represents the data being sent to the cell tower. On top of that, different frequencies serve different purposes. Higher frequency bands travel faster, but only for a limited range, and are unable to penetrate barriers (buildings, hills, trees, etc.) very well. Lower frequency bands can travel farther and through barriers well but operate at slower speeds. So higher frequency bands are better suited for higher populated urban areas whereas lower frequency bands work well in more rural environments.
Looking at this, cellular carriers (AT&T, Verizon, T-Mobile, etc.) would want to be able to provide access to multiple different types of bands so that their user can optimally access the internet in as many places as possible. With a limited amount of radio spectrum and technological capacity, though, this makes the process a little more complex.
Cellular Frequency Bands: A Closer Look
Frequency bands in the United States are licensed to different carriers through the Federal Communication Commission (FCC). While some bands are solely licensed to a specific carrier, most bands are split up into different “blocks” that each carrier can use. This way, multiple carriers can have access to bands that operate at certain frequencies and provide better performance. Each block consists of a group of one or more channels. A channel is a range of frequencies. Now I know we said bands were a range of frequencies, so what is the difference? Well, at a high level, cellular frequency bands represent a range of frequencies used to send data. But digging deeper, channels are the range of frequencies that live underneath the umbrella of bands.
The true definition of a band is that it is one or more similar channels that are grouped together and labeled as one band for convenience and licensing. These channels have a certain width, which determines the bandwidth, and the frequencies they cover can overlap with other channels in the band. From here, to allow multiple carriers, a band is broken up into different blocks, all containing those same channels, so that more carriers can use the band without interference.
To visualize how this works, let us look at the figure of a town community below. In this community we see a central road and two neighborhoods containing three loops of different houses that are of varying sizes.
Radio Spectrum = Central Road
-
The central road represents the radio spectrum that consists of all the different frequencies of waves. This spectrum gets sliced into different “neighborhoods” or frequency bands.
Neighborhood = Frequency Band
-
The neighborhood represents a frequency band. It takes up a specific amount of distance along the road, which is a specific range of frequencies.
Loops = Blocks
-
Each loop can be thought of as a different block. The blocks have the same houses within them and act as subdivisions of the neighborhood. Note that frequency bands are not required to have blocks, and that some frequency bands do not have blocks.
Houses = Channels
-
The houses represent channels. Each channel contains frequencies within the band’s specified range.
House Size = Bandwidth
-
The size of the house, or channel, can be thought of as the bandwidth. Notice how house 2 in the first neighborhood is bigger than house 1. This would mean this channel’s bandwidth is higher. Think about how with house 2, more people (or data) can fit in it, and by doing that, faster speeds result.
-
To increase bandwidth further, a method called carrier aggregation was developed. This allowed up to eight channels to be merged to support an even wider range of frequencies and reach peak bandwidths.
With this visual, we can see what frequency bands are and how they are organized in the radio spectrum. But a few questions/uncertainties might still be lingering. For instance, why do signals travel in a range of frequency? Also, where does 5G (and the other technologies for that matter) factor in?
Data Modulation
As we have now learned, frequency bands contain different channels that support a range of frequencies and that range represents the channel’s bandwidth. But why exactly does each channel need a range of frequencies to send a message? This is because when sending data, the signal wave undergoes a process called data modulation. Data is represented in binary code, which is a list of 0’s and 1’s. So, to translate that to a frequency wave that can be sent to a cell tower, the device uses a base “carrier” wave and adjusts it. By changing the frequency when a binary 1 is present, the wave now can carry the binary message that the cell tower can actually read and convert back to a digital signal. The main goal of data modulation is to squeeze as much data into the limited bandwidth present in the channels. Below is a figure that shows a form of digital modulation called Frequency Shift Keying (FSK).
In phones today an optimized process of modulation, combined with multiplexing, is used, called Orthogonal Frequency Division Multiplexing (OFDM). This process involves creating sub-channels within the channel, with each having its own sub-carrier waves to send the signal. These sub-carrier waves are positioned orthogonally from each other to avoid interference. This method creates a very high spectral efficiency and minimizes signal loss.
Where does 5G come in?
5G technology comes into play at the channel level and works to increase the bandwidth of those channels. 4G technologies normally operate with 20MHz wide channels, and 5G manages to handle 100MHz to 400MHz wide channels. Even more, 5G utilizes more advanced communication protocols that lowers latency—the time it takes for a request to be sent to a server and come back—down from ~53 milliseconds with 4G technology to below 30 milliseconds with 5G. Also factoring into 5G’s lower latency is its incorporation of channels with much higher frequencies than 4G ever had. While 4G technology supported channels in the range of 700-2500MHz, 5G technology is adopting frequency bands in the 25+ GHz range (as well as still having the lower bands present in 4G). Overall, with faster data and more bandwidth, 5G can serve more users quicker, which is something more and more needed as people continue to use more and more IOT devices. With 4G technology, roughly 4,000 devices can be supported per square kilometer, but with 5G technology 1 million devices per square kilometer can be connected.
Tying it all Together
Using this information, we can see the obstacles that Mark Wells indicates in the episode. Smartphones have access to lots of different frequency bands to try and connect to cell towers. They can even “roam” and use other carrier’s blocks to try and connect. Mobile IOT devices, such as the solutions provided by Positioning Universal, only have access to one or maybe a few frequency bands and only one technology (4G LTE). Since different frequencies work better in different geographies/environments, this poses a big connectivity challenge. However, Positioning Universal thrives on working through this limitation and still providing exceptional connectivity and real-time speeds. Every product undergoes thousands of miles of road testing in many different environments and geographies. Also, Positioning Universal offers unparalleled cloud-based device management. By diligently handling all over-the-air programming of deployed devices—including software updates and settings changes—each device reliably connects and delivers all of its critical information no matter what condition.